Stormwater Best Management Practices in an Ultra-Urban Setting: Selection and Monitoring
Monitoring Case Study-Compost Stormwater Treatment System, Hillsboro, Oregon
This case study is based on an evaluation of a leaf compost filter system. The initial evaluation of the system was reported by Stewart (1992), with a summary three-year evaluation of the same site reported by Stormwater Management (1994).
A prototype of a compost stormwater treatment facility (CSF) was constructed at southwest 185th Avenue in Washington County, Oregon. Southwest 185th Avenue was widened in 1987 to five lanes with additional bike lanes and sidewalks. As part of this road widening, a relatively narrow water quality swale was constructed downstream from a storm runoff outlet. The prototype CSF was constructed at the head of the swale, with the swale (1.8 m [6 ft] wide and 76.3 m [250 ft] long) draining into a wetland mitigation pond, and then into Beaverton Creek. The CSF was constructed to treat runoff from 30 ha (74 ac) that drained to the existing water quality swale via the stormwater pipe. The area that drains to the site consists of 1.6 ha (3.9 ac) of five-lane arterial and 28.4 ha (70.1 ac) of mixed residential land use. Because it is widely reported that high-quality composts have a very high capacity for adsorbing heavy metals, oils, greases, nutrients, and organic toxins, compost was used as a filter medium for this test facility. Construction of the full-scale prototype was completed in August 1991. Funding was provided by the Unified Sewerage Agency (USA) to evaluate the performance of the CSF for three years.
Study Objective
Design and Operation
The CSF was sized primarily based on the results of pilot study permeability tests, which were conducted prior to the start of construction. Due to its high quality, Portland leaf compost was selected for the filter medium. The results of the permeability tests using the Portland leaf compost mixture produced an average flow rate of 91.6 Lpm/m2 (2.25 gpm/ft2) for the first half hour of flow through a drained compost bed. This is equivalent to a compost bed surface area requirement of 656 m2/m3/s (200 ft2/cfs) of flow. The peak design flow for the drainage basin was estimated using the rational method. For this basin, the peak design flow for the test facility was determined to be 0.2 m3/s (6.7 cfs). According to this peak flow rate, the treatment facility would require 124.6 m2 (1,340 ft2) of compost bed surface area. Due to site restrictions, the CSF was downsized by approximately 10 percent to accommodate a bed surface area of 111.6 m2 (1,200 ft2). The construction bid price was $12,500, with only minor problems and clarifications needed. The prototype filter required 91.8 m3 (120 yd3) of leaf compost, at a delivered cost of $1,200.
The compost prototype test facility was designed to be retrofitted into the existing stormwater runoff quality swale of southwest 185th Avenue. To accommodate the compost system, the existing swale was widened to 3.1 m (10 ft) and given a 2:1 side slope. The longitudinal slope of the swale was graded at 2 percent from the influent to the effluent end. A typical cross section of the compost treatment system is shown in Figure 58. A polyethylene liner was installed along its length to prevent percolation of stormwater into the ground and to minimize effluent loss for monitoring purposes. A riprap geotextile fabric was installed over this liner to minimize tearing of the polyethylene liner during construction. Two 100 mm (4 in) perforated PVC drain pipes were installed along the entire length of the compost facility to facilitate drainage through the system and provide an effluent sampling point for monitoring.
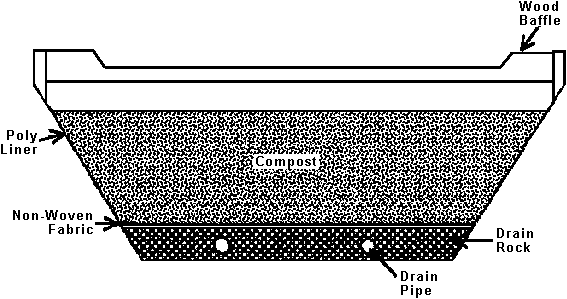
The original design called for the drain pipes to be covered with a 15.2 cm (6 in) layer of 5.1 cm (2 in) washed drain rock and an additional 5.1 cm (2 in) layer of pea gravel to provide the underdrain system. After a nonwoven filter fabric was secured on top of the drain rock (see Figure 58), a 45.7 cm (18 in) layer of 1.9 cm (3/4 in) Portland leaf compost was placed over the nonwoven filter fabric. However, at the time of construction, the washed 5.1 cm (2 in) drain rock was not available and a substitute nonwashed 5.1 cm (2 in) drain rock was used in its place. It became apparent to USA that the dirt on the nonwashed drain rock would affect the sampling program results. The USA laboratory obtained samples of the rock and conducted tests in which the samples were washed and analyzed. The washings contained high levels of total suspended solids (TSS), total phosphorus, aluminum, barium, cobalt, chromium, copper, iron, nickel, and vanadium. As a result, tests of effluent from the first few storms probably contained contaminants contributed by the drain rock.
The compost filter system is divided into two 15.3 m (50 ft) cells that are 3.66 m (12 ft) wide at the surface. A layer of riprap was placed over the compost next to the wooden barriers in Cells 1 and 2 to distribute the flow coming over the wood barriers and to prevent washing out the compost next to the barriers. (Figure 59 shows a plan view of the compost filter system with the wooden barriers.) Unfortunately, an excessive amount of riprap was placed, causing the underlying compost to settle rapidly. The riprap also caused the stormwater flow to create small channels in the medium and flow through an isolated section of the compost bed. This problem was rectified by removing the majority of the riprap that had been placed beneath the wooden barriers.
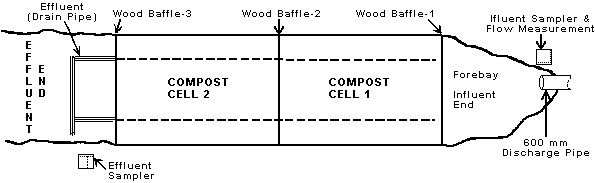
The operation of the filter begins at an existing 600 mm (24 in) stormwater pipe that discharges into a forebay area prior to entering the compost filter system (see Figure 59). The cells are subdivided using wooden baffles to guide the flow as it leaves the forebay area. Wood baffle No. 1 is entrenched into the ground so all of the stormwater passes from the forebay area into the compost filter system. Wood baffles No. 2 and No. 3 have 5.1 cm (2 in) gaps at their bases to allow free drainage of treated stormwater through the rock underdrain and out to the effluent end (see Figure 60). The effluent ends of the two 100 mm (4 in) parallel drain pipes were coupled to provide for a single effluent sampling point for monitoring purposes (see Figure 59).
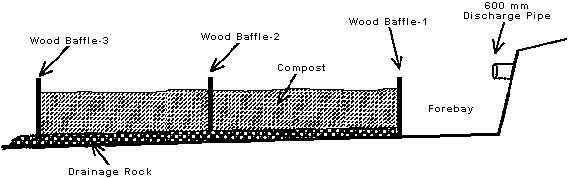
Monitoring
The monitoring program for the newly constructed compost facility began in August 1991. Sampling and laboratory analyses were conducted by USA using standard USEPA sampling protocols. A sampling of 1/37,850 L (1/10,000 gal) was based on rainfall of 5 mm (0.2 in) and the size of the drainage basin. One sampler was located at the influent end and the other at the effluent end. The monitoring program included both first-flush and flow-paced sampling, using programmable automatic samplers. First-flush runoff represents the most critical need for stormwater treatment due to the effect of high pollutant loadings on receiving waters. The first-flush portion (first half hour) of a storm event carries a significantly higher pollutant loading than the remaining or flow-paced portion. The automatic sampler was programmed so that when the flow meter detected a flow depth of 6.1 cm (0.2 ft) in the discharge pipe, it was set to trigger both samplers to take two time-paced (first-flush) samples (with a 10-min delay for the effluent sampler). The automatic sampler then switched to flow-paced or weighted composite sampling. Each time the flow meter sensed 3,785 L (100 gal), it triggered a flow pulse to the samplers. The samples were collected following cessation of each sampled storm event.
During the first year of testing (1991-1992), a total of seven storm events were sampled. A total of eight storm events, four for each year, were sampled during the second (1992-1993) and the third years (1993-1994) of testing. The results of three years of monitoring are given in Table 35. Table 35 presents average mass balance pollutant removal efficiencies for both first flush and combined first flush and flow paced testing. A blank entry indicates that data was not available or not collected.
Table 35. Mass balance pollutant removal efficiencies for three seasons of testing (%)
Parameter |
1991-92 |
1992-93 |
1993-94 |
Turbidity
Combined
First Flush |
84.2
93.4 |
78.4
85.3 |
78.4
81.4 |
TSS
Combined
First Flush |
94.8
98.3 |
88.5
91.4 |
86.0
89.0 |
COD
Combined
First Flush |
66.9
89.5 |
76.3
82.1 |
74.0
79.8 |
Total P
Combined
First Flush |
40.5
67.3 |
53.2
68.9 |
65.5
72.9 |
TKN
Combined
First Flush |
55.9
84.0 |
50.5
60.8 |
66.7
69.0 |
Fe
Combined
First Flush |
89.0
94.0 |
95.5
97.5 |
79.6
82.9 |
Cr
Combined
First Flush |
61.2
92.4 |
74.5
80.8 |
64.3
72.8 |
Cu
Combined
First Flush |
66.7
83.7 |
63.5
73.9 |
64.1
70.7 |
Pb
Combined
First Flush |
N/A |
85.1
89.0 |
81.4
84.0 |
Zn
Combined
First Flush |
88.3
92.8 |
75.8
83.1 |
79.9
83.1 |
Conclusions
-
The CSF operated very effectively in the first three seasons of operation, with overall mass balance pollutant removals averaging 81 percent for oils and grease, 84 percent for petroleum hydrocarbons, 58 to 94 percent for solids and nutrients, and 68 to 93 percent for metals.
-
Although nutrient removals were not as good as expected, the removal of total phosphorus was 65.5 percent by the third season.
-
First-flush removal rates were significantly higher than flow-paced removal rates for all of the pollutants and years tested. This would tend to indicate that the system operates most efficiently above some threshold pollutant load that is closely related to the first half hour of stormwater runoff.
-
Results of the monitoring indicate that the CSF shows particular promise for treating heavily polluted stormwater runoff from industrial areas, heavily traveled urban streets, waste transfer stations, airports, and parking lots. In addition, the CSF design permits existing water quality swales to be retrofitted to improve pollutant removal rates. Unlike water quality swales that are not designed to retain stormwater runoff and tend to allow intense influent flows to pass over without proper treatment, a properly sized CSF will retain and treat the design storm runoff.
-
Although the peak design storm never occurred during the testing period, the system performed very efficiently for all of the storm events that it treated.
-
Future specifications should require double-washed drain rock with no exceptions.
-
In future designs, more efficient horizontal flow distribution can be accomplished by using flat-plate notched weirs, bolted to the wooden barriers, and setting the first two wooden barriers closer together to form a more effective ponding section.
References
Stewart, W.S. 1992. Compost Storm Water Treatment System. W&H Pacific Consultants, Portland, OR. Final Report.
Stormwater Management. 1994. Three Year Performance Summary of Stormwater Pollutant and Treatment - 185th Avenue, Hillsboro, Oregon. Technical Memorandum. Stormwater Management, Portland, OR.
|